Cap-dependent deadenylation of mRNA
- PMID: 10698948
- PMCID: PMC305646
- DOI: 10.1093/emboj/19.5.1079
Cap-dependent deadenylation of mRNA
Abstract
Poly(A) tail removal is often the initial and rate-limiting step in mRNA decay and is also responsible for translational silencing of maternal mRNAs during oocyte maturation and early development. Here we report that deadenylation in HeLa cell extracts and by a purified mammalian poly(A)-specific exoribonuclease, PARN (previously designated deadenylating nuclease, DAN), is stimulated by the presence of an m(7)-guanosine cap on substrate RNAs. Known cap-binding proteins, such as eIF4E and the nuclear cap-binding complex, are not detectable in the enzyme preparation, and PARN itself binds to m(7)GTP-Sepharose and is eluted specifically with the cap analog m(7)GTP. Xenopus PARN is known to catalyze mRNA deadenylation during oocyte maturation. The enzyme is depleted from oocyte extract with m(7)GTP-Sepharose, can be photocross-linked to the m(7)GpppG cap and deadenylates m(7)GpppG-capped RNAs more efficiently than ApppG-capped RNAs both in vitro and in vivo. These data provide additional evidence that PARN is responsible for deadenylation during oocyte maturation and suggest that interactions between 5' cap and 3' poly(A) tail may integrate translational efficiency with mRNA stability.
Figures
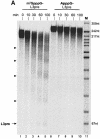
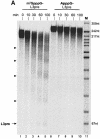
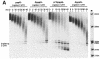
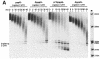
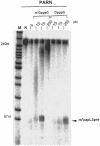
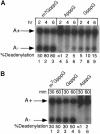
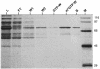
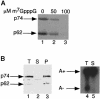
Similar articles
-
Structural basis of m(7)GpppG binding to poly(A)-specific ribonuclease.Structure. 2009 Feb 13;17(2):276-86. doi: 10.1016/j.str.2008.11.012. Structure. 2009. PMID: 19217398
-
Crystal structure of the RRM domain of poly(A)-specific ribonuclease reveals a novel m(7)G-cap-binding mode.J Mol Biol. 2008 Oct 17;382(4):827-34. doi: 10.1016/j.jmb.2008.07.073. Epub 2008 Jul 31. J Mol Biol. 2008. PMID: 18694759
-
Poly(A)-specific ribonuclease (PARN): an allosterically regulated, processive and mRNA cap-interacting deadenylase.Crit Rev Biochem Mol Biol. 2013 Mar-Apr;48(2):192-209. doi: 10.3109/10409238.2013.771132. Epub 2013 Mar 15. Crit Rev Biochem Mol Biol. 2013. PMID: 23496118 Review.
-
Allosteric regulation of human poly(A)-specific ribonuclease by cap and potassium ions.Biochem Biophys Res Commun. 2009 Feb 6;379(2):341-5. doi: 10.1016/j.bbrc.2008.12.056. Epub 2008 Dec 25. Biochem Biophys Res Commun. 2009. PMID: 19103158
-
Messenger RNA turnover in eukaryotes: pathways and enzymes.Crit Rev Biochem Mol Biol. 2004 Jul-Aug;39(4):197-216. doi: 10.1080/10409230490513991. Crit Rev Biochem Mol Biol. 2004. PMID: 15596551 Review.
Cited by
-
mRNA decay enzymes: decappers conserved between yeast and mammals.Proc Natl Acad Sci U S A. 2002 Oct 1;99(20):12512-4. doi: 10.1073/pnas.212518099. Epub 2002 Sep 23. Proc Natl Acad Sci U S A. 2002. PMID: 12271148 Free PMC article. No abstract available.
-
Modulation of enteroviral proteinase cleavage of poly(A)-binding protein (PABP) by conformation and PABP-associated factors.Virology. 2008 May 25;375(1):59-72. doi: 10.1016/j.virol.2008.02.002. Epub 2008 Mar 5. Virology. 2008. PMID: 18321554 Free PMC article.
-
Identification of PARN nuclease activity inhibitors by computational-based docking and high-throughput screening.Sci Rep. 2023 Mar 31;13(1):5244. doi: 10.1038/s41598-023-32039-z. Sci Rep. 2023. PMID: 37002320 Free PMC article.
-
The PARN deadenylase targets a discrete set of mRNAs for decay and regulates cell motility in mouse myoblasts.PLoS Genet. 2012;8(8):e1002901. doi: 10.1371/journal.pgen.1002901. Epub 2012 Aug 30. PLoS Genet. 2012. PMID: 22956911 Free PMC article.
-
Poly(A)-specific ribonuclease deficiency impacts telomere biology and causes dyskeratosis congenita.J Clin Invest. 2015 May;125(5):2151-60. doi: 10.1172/JCI78963. Epub 2015 Apr 20. J Clin Invest. 2015. PMID: 25893599 Free PMC article.
References
Publication types
MeSH terms
Substances
LinkOut - more resources
Full Text Sources
Other Literature Sources
Molecular Biology Databases
Miscellaneous