Poly(A)-specific ribonuclease deficiency impacts telomere biology and causes dyskeratosis congenita
- PMID: 25893599
- PMCID: PMC4463202
- DOI: 10.1172/JCI78963
Poly(A)-specific ribonuclease deficiency impacts telomere biology and causes dyskeratosis congenita
Abstract
Dyskeratosis congenita (DC) and related syndromes are inherited, life-threatening bone marrow (BM) failure disorders, and approximately 40% of cases are currently uncharacterized at the genetic level. Here, using whole exome sequencing (WES), we have identified biallelic mutations in the gene encoding poly(A)-specific ribonuclease (PARN) in 3 families with individuals exhibiting severe DC. PARN is an extensively characterized exonuclease with deadenylation activity that controls mRNA stability in part and therefore regulates expression of a large number of genes. The DC-associated mutations identified affect key domains within the protein, and evaluation of patient cells revealed reduced deadenylation activity. This deadenylation deficiency caused an early DNA damage response in terms of nuclear p53 regulation, cell-cycle arrest, and reduced cell viability upon UV treatment. Individuals with biallelic PARN mutations and PARN-depleted cells exhibited reduced RNA levels for several key genes that are associated with telomere biology, specifically TERC, DKC1, RTEL1, and TERF1. Moreover, PARN-deficient cells also possessed critically short telomeres. Collectively, these results identify a role for PARN in telomere maintenance and demonstrate that it is a disease-causing gene in a subset of patients with severe DC.
Figures
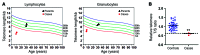
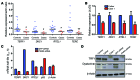
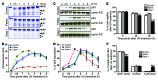
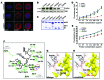
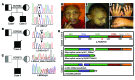
Comment in
-
mRNA deadenylation and telomere disease.J Clin Invest. 2015 May;125(5):1796-8. doi: 10.1172/JCI81506. Epub 2015 Apr 20. J Clin Invest. 2015. PMID: 25893598 Free PMC article.
-
Long tails, short telomeres: Dyskeratosis congenita.Oncotarget. 2015 Jun 10;6(16):13856-7. doi: 10.18632/oncotarget.4388. Oncotarget. 2015. PMID: 26116823 Free PMC article. No abstract available.
Similar articles
-
mRNA deadenylation and telomere disease.J Clin Invest. 2015 May;125(5):1796-8. doi: 10.1172/JCI81506. Epub 2015 Apr 20. J Clin Invest. 2015. PMID: 25893598 Free PMC article.
-
Bone marrow failure and developmental delay caused by mutations in poly(A)-specific ribonuclease (PARN).J Med Genet. 2015 Nov;52(11):738-48. doi: 10.1136/jmedgenet-2015-103292. Epub 2015 Sep 4. J Med Genet. 2015. PMID: 26342108
-
From incomplete penetrance with normal telomere length to severe disease and telomere shortening in a family with monoallelic and biallelic PARN pathogenic variants.Hum Mutat. 2019 Dec;40(12):2414-2429. doi: 10.1002/humu.23898. Epub 2019 Sep 15. Hum Mutat. 2019. PMID: 31448843 Free PMC article.
-
Poly (A)-specific ribonuclease (PARN): More than just "mRNA stock clearing".Life Sci. 2021 Nov 15;285:119953. doi: 10.1016/j.lfs.2021.119953. Epub 2021 Sep 11. Life Sci. 2021. PMID: 34520768 Review.
-
The molecular genetics of the telomere biology disorders.RNA Biol. 2016 Aug 2;13(8):696-706. doi: 10.1080/15476286.2015.1094596. Epub 2015 Sep 23. RNA Biol. 2016. PMID: 26400640 Free PMC article. Review.
Cited by
-
The short and long telomere syndromes: paired paradigms for molecular medicine.Curr Opin Genet Dev. 2015 Aug;33:1-9. doi: 10.1016/j.gde.2015.06.004. Epub 2015 Jul 29. Curr Opin Genet Dev. 2015. PMID: 26232116 Free PMC article. Review.
-
Pre-Ribosomal RNA Processing in Human Cells: From Mechanisms to Congenital Diseases.Biomolecules. 2018 Oct 24;8(4):123. doi: 10.3390/biom8040123. Biomolecules. 2018. PMID: 30356013 Free PMC article. Review.
-
Inhibition of telomerase RNA decay rescues telomerase deficiency caused by dyskerin or PARN defects.Nat Struct Mol Biol. 2016 Apr;23(4):286-92. doi: 10.1038/nsmb.3184. Epub 2016 Mar 7. Nat Struct Mol Biol. 2016. PMID: 26950371 Free PMC article.
-
Genetics in Idiopathic Pulmonary Fibrosis Pathogenesis, Prognosis, and Treatment.Front Med (Lausanne). 2017 Sep 25;4:154. doi: 10.3389/fmed.2017.00154. eCollection 2017. Front Med (Lausanne). 2017. PMID: 28993806 Free PMC article. Review.
-
Multiple bilateral hip fractures in a patient with dyskeratosis congenita caused by a novel mutation in the PARN gene.Osteoporos Int. 2021 Jun;32(6):1227-1231. doi: 10.1007/s00198-020-05758-6. Epub 2020 Nov 27. Osteoporos Int. 2021. PMID: 33244623
References
Publication types
MeSH terms
Substances
Grants and funding
LinkOut - more resources
Full Text Sources
Other Literature Sources
Molecular Biology Databases
Research Materials
Miscellaneous