Structural basis for the ligand recognition and signaling of free fatty acid receptors
- PMID: 38198545
- PMCID: PMC10780892
- DOI: 10.1126/sciadv.adj2384
Structural basis for the ligand recognition and signaling of free fatty acid receptors
Abstract
Free fatty acid receptors 1 to 4 (FFA1 to FFA4) are class A G protein-coupled receptors (GPCRs). FFA1 to FFA3 share substantial sequence similarity, whereas FFA4 is unrelated. However, FFA1 and FFA4 are activated by long-chain fatty acids, while FFA2 and FFA3 respond to short-chain fatty acids generated by intestinal microbiota. FFA1, FFA2, and FFA4 are potential drug targets for metabolic and inflammatory conditions. Here, we determined the active structures of FFA1 and FFA4 bound to docosahexaenoic acid, FFA4 bound to the synthetic agonist TUG-891, and butyrate-bound FFA2, each complexed with an engineered heterotrimeric Gq protein (miniGq), by cryo-electron microscopy. Together with computational simulations and mutagenesis studies, we elucidated the similarities and differences in the binding modes of fatty acid ligands to their respective GPCRs. Our findings unveiled distinct mechanisms of receptor activation and G protein coupling. We anticipate that these outcomes will facilitate structure-based drug development and underpin future research on this group of GPCRs.
Figures
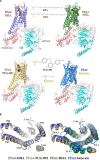
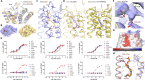
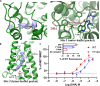
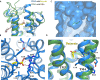
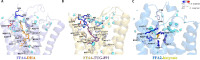
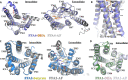
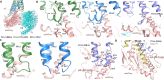
Update of
-
Structural basis for the ligand recognition and signaling of free fatty acid receptors.bioRxiv [Preprint]. 2023 Aug 21:2023.08.20.553924. doi: 10.1101/2023.08.20.553924. bioRxiv. 2023. Update in: Sci Adv. 2024 Jan 12;10(2):eadj2384. doi: 10.1126/sciadv.adj2384. PMID: 37662198 Free PMC article. Updated. Preprint.
Similar articles
-
Structural basis for the ligand recognition and signaling of free fatty acid receptors.bioRxiv [Preprint]. 2023 Aug 21:2023.08.20.553924. doi: 10.1101/2023.08.20.553924. bioRxiv. 2023. Update in: Sci Adv. 2024 Jan 12;10(2):eadj2384. doi: 10.1126/sciadv.adj2384. PMID: 37662198 Free PMC article. Updated. Preprint.
-
Application of GPCR Structures for Modelling of Free Fatty Acid Receptors.Handb Exp Pharmacol. 2017;236:57-77. doi: 10.1007/164_2016_52. Handb Exp Pharmacol. 2017. PMID: 27757764
-
Characterizing pharmacological ligands to study the long-chain fatty acid receptors GPR40/FFA1 and GPR120/FFA4.Br J Pharmacol. 2015 Jul;172(13):3254-65. doi: 10.1111/bph.12879. Epub 2015 Feb 27. Br J Pharmacol. 2015. PMID: 25131623 Free PMC article. Review.
-
Free-fatty acid receptor-4 (GPR120): Cellular and molecular function and its role in metabolic disorders.Biochem Pharmacol. 2016 Jun 15;110-111:1-15. doi: 10.1016/j.bcp.2016.01.021. Epub 2016 Jan 28. Biochem Pharmacol. 2016. PMID: 26827942 Free PMC article. Review.
-
Enteroendocrine cell-derived peptide YY signalling is stimulated by pinolenic acid or Intralipid and involves coactivation of fatty acid receptors FFA1, FFA4 and GPR119.Neuropeptides. 2024 Dec;108:102477. doi: 10.1016/j.npep.2024.102477. Epub 2024 Oct 11. Neuropeptides. 2024. PMID: 39427565
Cited by
-
Novel Phenoxyalkanoic Acid Derivatives as Free Fatty Acid Receptor 4 Agonists for Treating Type 2 Diabetes Mellitus.Int J Mol Sci. 2024 Oct 25;25(21):11476. doi: 10.3390/ijms252111476. Int J Mol Sci. 2024. PMID: 39519029 Free PMC article.
References
-
- Alvarez-Curto E., Milligan G., Metabolism meets immunity: The role of free fatty acid receptors in the immune system. Biochem. Pharmacol. 114, 3–13 (2016). - PubMed
-
- Kimura I., Ichimura A., Ohue-Kitano R., Igarashi M., Free fatty acid receptors in health and disease. Physiol. Rev. 100, 171–210 (2020). - PubMed
-
- Briscoe C. P., Tadayyon M., Andrews J. L., Benson W. G., Chambers J. K., Eilert M. M., Ellis C., Elshourbagy N. A., Goetz A. S., Minnick D. T., Murdock P. R., Sauls H. R. Jr., Shabon U., Spinage L. D., Strum J. C., Szekeres P. G., Tan K. B., Way J. M., Ignar D. M., Wilson S., Muir A. I., The orphan G protein-coupled receptor GPR40 is activated by medium and long chain fatty acids. J. Biol. Chem. 278, 11303–11311 (2003). - PubMed
-
- McCarville J. L., Chen G. Y., Cuevas V. D., Troha K., Ayres J. S., Microbiota metabolites in health and disease. Annu. Rev. Immunol. 38, 147–170 (2020). - PubMed