Free fatty acid receptor 4 responds to endogenous fatty acids to protect the heart from pressure overload
- PMID: 33752243
- PMCID: PMC8930069
- DOI: 10.1093/cvr/cvab111
Free fatty acid receptor 4 responds to endogenous fatty acids to protect the heart from pressure overload
Abstract
Aims: Free fatty acid receptor 4 (Ffar4) is a G-protein-coupled receptor for endogenous medium-/long-chain fatty acids that attenuates metabolic disease and inflammation. However, the function of Ffar4 in the heart is unclear. Given its putative beneficial role, we hypothesized that Ffar4 would protect the heart from pathologic stress.
Methods and results: In mice lacking Ffar4 (Ffar4KO), we found that Ffar4 is required for an adaptive response to pressure overload induced by transverse aortic constriction (TAC), identifying a novel cardioprotective function for Ffar4. Following TAC, remodelling was worsened in Ffar4KO hearts, with greater hypertrophy and contractile dysfunction. Transcriptome analysis 3-day post-TAC identified transcriptional deficits in genes associated with cytoplasmic phospholipase A2α signalling and oxylipin synthesis and the reduction of oxidative stress in Ffar4KO myocytes. In cultured adult cardiac myocytes, Ffar4 induced the production of the eicosapentaenoic acid (EPA)-derived, pro-resolving oxylipin 18-hydroxyeicosapentaenoic acid (18-HEPE). Furthermore, the activation of Ffar4 attenuated cardiac myocyte death from oxidative stress, while 18-HEPE rescued Ffar4KO myocytes. Systemically, Ffar4 maintained pro-resolving oxylipins and attenuated autoxidation basally, and increased pro-inflammatory and pro-resolving oxylipins, including 18-HEPE, in high-density lipoproteins post-TAC. In humans, Ffar4 expression decreased in heart failure, while the signalling-deficient Ffar4 R270H polymorphism correlated with eccentric remodelling in a large clinical cohort paralleling changes observed in Ffar4KO mice post-TAC.
Conclusion: Our data indicate that Ffar4 in cardiac myocytes responds to endogenous fatty acids, reducing oxidative injury, and protecting the heart from pathologic stress, with significant translational implications for targeting Ffar4 in cardiovascular disease.
Keywords: 18-hydroxyeicosapentaenoic acid (18-HEPE); Cytoplasmic phospholipase A2α (cPLA2α); Eicosapentaenoic acid (EPA); Free fatty acid receptor 4 (Ffar4); GPR120; Heart failure.
Published on behalf of the European Society of Cardiology. All rights reserved. © The Author(s) 2021. For permissions, please email: [email protected].
Figures
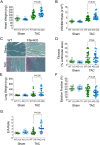
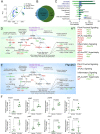
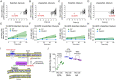
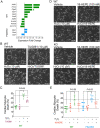
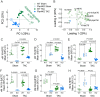
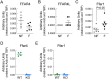
Similar articles
-
FFAR4: A New Player in Cardiometabolic Disease?Endocrinology. 2021 Aug 1;162(8):bqab111. doi: 10.1210/endocr/bqab111. Endocrinology. 2021. PMID: 34043793 Free PMC article. Review.
-
FFAR4 regulates cardiac oxylipin balance to promote inflammation resolution in HFpEF secondary to metabolic syndrome.J Lipid Res. 2023 Jun;64(6):100374. doi: 10.1016/j.jlr.2023.100374. Epub 2023 Apr 17. J Lipid Res. 2023. PMID: 37075982 Free PMC article.
-
Free fatty acid receptor 4 in cardiac myocytes ameliorates ischemic cardiomyopathy.bioRxiv [Preprint]. 2024 Apr 15:2024.04.12.589280. doi: 10.1101/2024.04.12.589280. bioRxiv. 2024. PMID: 38659901 Free PMC article. Preprint.
-
Docosahexaenoic Acid Attenuates the Progression of Nonalcoholic Steatohepatitis by Suppressing the Adipocyte Inflammation via the G Protein-Coupled Receptor 120/Free Fatty Acid Receptor 4 Pathway.Pharmacology. 2022;107(5-6):330-338. doi: 10.1159/000522117. Epub 2022 Feb 21. Pharmacology. 2022. PMID: 35189618
-
ω3-Polyunsaturated fatty acids for heart failure: Effects of dose on efficacy and novel signaling through free fatty acid receptor 4.J Mol Cell Cardiol. 2017 Feb;103:74-92. doi: 10.1016/j.yjmcc.2016.12.003. Epub 2016 Dec 14. J Mol Cell Cardiol. 2017. PMID: 27986444 Free PMC article. Review.
Cited by
-
Eicosapentaenoic Acid (EPA) and Docosahexaenoic Acid (DHA) Ameliorate Heart Failure through Reductions in Oxidative Stress: A Systematic Review and Meta-Analysis.Antioxidants (Basel). 2024 Aug 6;13(8):955. doi: 10.3390/antiox13080955. Antioxidants (Basel). 2024. PMID: 39199201 Free PMC article. Review.
-
Mitophagy: A Potential Target for Pressure Overload-Induced Cardiac Remodelling.Oxid Med Cell Longev. 2022 Sep 27;2022:2849985. doi: 10.1155/2022/2849985. eCollection 2022. Oxid Med Cell Longev. 2022. PMID: 36204518 Free PMC article. Review.
-
Apelin receptor inhibition in ischemia-reperfused mouse hearts protected by endogenous n-3 polyunsaturated fatty acids.Front Pharmacol. 2023 Oct 24;14:1145413. doi: 10.3389/fphar.2023.1145413. eCollection 2023. Front Pharmacol. 2023. PMID: 37942483 Free PMC article.
-
FFAR4: A New Player in Cardiometabolic Disease?Endocrinology. 2021 Aug 1;162(8):bqab111. doi: 10.1210/endocr/bqab111. Endocrinology. 2021. PMID: 34043793 Free PMC article. Review.
-
New Progress in the Molecular Regulations and Therapeutic Applications in Cardiac Oxidative Damage Caused by Pressure Overload.Antioxidants (Basel). 2022 Apr 29;11(5):877. doi: 10.3390/antiox11050877. Antioxidants (Basel). 2022. PMID: 35624741 Free PMC article. Review.
References
-
- Alvarez-Curto E, Milligan G.. Metabolism meets immunity: the role of free fatty acid receptors in the immune system. Biochem Pharmacol 2016;114:3–13. - PubMed
-
- Christiansen E, Watterson KR, Stocker CJ, Sokol E, Jenkins L, Simon K, Grundmann M, Petersen RK, Wargent ET, Hudson BD, Kostenis E, Ejsing CS, Cawthorne MA, Milligan G, Ulven T.. Activity of dietary fatty acids on FFA1 and FFA4 and characterisation of pinolenic acid as a dual FFA1/FFA4 agonist with potential effect against metabolic diseases. Br J Nutr 2015;113:1677–1688. - PubMed
Publication types
MeSH terms
Substances
Grants and funding
LinkOut - more resources
Full Text Sources
Other Literature Sources
Medical
Research Materials