Novel GPR120 agonist TUG891 modulates fat taste perception and preference and activates tongue-brain-gut axis in mice
- PMID: 31806728
- PMCID: PMC6997603
- DOI: 10.1194/jlr.RA119000142
Novel GPR120 agonist TUG891 modulates fat taste perception and preference and activates tongue-brain-gut axis in mice
Abstract
GPR120 is implicated as a lipid receptor in the oro-sensory detection of dietary fatty acids. However, the effects of GPR120 activation on dietary fat intake or obesity are not clearly understood. We investigated to determine whether the binding of TUG891, a novel GPR120 agonist, to lingual GPR120 modulates fat preference in mice. We explored the effects of TUG891 on obesity-related hormones and conducted behavioral choice tests on mice to better understand the physiologic relevance of the action of TUG891. In cultured mouse and human taste bud cells (TBCs), TUG891 induced a rapid increase in Ca2+ by acting on GPR120. A long-chain dietary fatty acid, linoleic acid (LA), also recruited Ca2+ via GPR120 in human and mouse TBCs. Both TUG891 and LA induced ERK1/2 phosphorylation and enhanced in vitro release of glucagon-like peptide-1 from cultured human and mouse TBCs. In situ application of TUG891 onto the tongue of anesthetized mice triggered the secretion of pancreatobiliary juice, probably via the tongue-brain-gut axis. Furthermore, lingual application of TUG891 altered circulating concentrations of cholecystokinin and adipokines, associated with decreased circulating LDL, in conscious mice. In behavioral tests, mice exhibited a spontaneous preference for solutions containing either TUG891 or LA instead of a control. However, addition of TUG891 to a solution containing LA significantly curtailed fatty acid preference. Our study demonstrates that TUG891 binds to lingual GPR120 receptors, activates the tongue-brain-gut axis, and modulates fat preference. These findings may support the development of new fat taste analogs that can change the approach to obesity prevention and treatment.
Keywords: extracellular signal-regulated kinase 1/2; glucagon-like peptide-1; linoleic acid; obesity.
Copyright © 2020 Murtaza et al.
Conflict of interest statement
The authors declare that they have no conflicts of interest with the contents of this article.
Figures
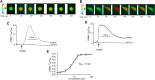
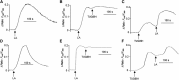
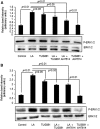
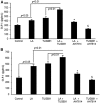
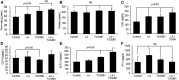
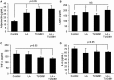
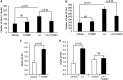
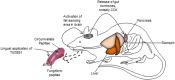
Similar articles
-
CD36- and GPR120-mediated Ca²⁺ signaling in human taste bud cells mediates differential responses to fatty acids and is altered in obese mice.Gastroenterology. 2014 Apr;146(4):995-1005. doi: 10.1053/j.gastro.2014.01.006. Epub 2014 Jan 9. Gastroenterology. 2014. PMID: 24412488 Free PMC article.
-
ERK1/2 activation in human taste bud cells regulates fatty acid signaling and gustatory perception of fat in mice and humans.FASEB J. 2016 Oct;30(10):3489-3500. doi: 10.1096/fj.201600422R. Epub 2016 Jun 29. FASEB J. 2016. PMID: 27358389 Free PMC article.
-
The oral lipid sensor GPR120 is not indispensable for the orosensory detection of dietary lipids in mice.J Lipid Res. 2015 Feb;56(2):369-78. doi: 10.1194/jlr.M055202. Epub 2014 Dec 8. J Lipid Res. 2015. PMID: 25489006 Free PMC article.
-
Ca2+ signaling in taste bud cells and spontaneous preference for fat: unresolved roles of CD36 and GPR120.Biochimie. 2014 Jan;96:8-13. doi: 10.1016/j.biochi.2013.06.005. Epub 2013 Jun 15. Biochimie. 2014. PMID: 23774298 Review.
-
GPR120 agonism as a countermeasure against metabolic diseases.Drug Discov Today. 2014 May;19(5):670-9. doi: 10.1016/j.drudis.2013.11.021. Epub 2013 Dec 4. Drug Discov Today. 2014. PMID: 24315954 Review.
Cited by
-
Huoxue Jiangtang Decoction Alleviates Type 2 Diabetes Mellitus by Regulating the Oral Microbiota and Food Preferences.Diabetes Metab Syndr Obes. 2022 Nov 30;15:3739-3751. doi: 10.2147/DMSO.S391226. eCollection 2022. Diabetes Metab Syndr Obes. 2022. PMID: 36474726 Free PMC article.
-
Adiponectin Signaling Modulates Fat Taste Responsiveness in Mice.Nutrients. 2024 Oct 30;16(21):3704. doi: 10.3390/nu16213704. Nutrients. 2024. PMID: 39519538 Free PMC article.
-
Physiology of the tongue with emphasis on taste transduction.Physiol Rev. 2023 Apr 1;103(2):1193-1246. doi: 10.1152/physrev.00012.2022. Epub 2022 Nov 24. Physiol Rev. 2023. PMID: 36422992 Free PMC article. Review.
-
Steviol rebaudiosides bind to four different sites of the human sweet taste receptor (T1R2/T1R3) complex explaining confusing experiments.Commun Chem. 2024 Oct 18;7(1):236. doi: 10.1038/s42004-024-01324-x. Commun Chem. 2024. PMID: 39424933 Free PMC article.
-
Cellular and Molecular Mechanisms of Fat Taste Perception.Handb Exp Pharmacol. 2022;275:247-270. doi: 10.1007/164_2021_437. Handb Exp Pharmacol. 2022. PMID: 33547589
References
-
- Besnard P., Passilly-Degrace P., and Khan N. A.. 2016. Taste of fat: a sixth taste modality? Physiol. Rev. 96: 151–176. - PubMed
-
- Keast R. S. J., and Costanzo A.. 2014. Is fat the sixth taste primary? Evidence and implications. Flavour. 4: 1–7.
-
- Ozdener M. H., Subramaniam S., Sundaresan S., Sery O., Hashimoto T., Asakawa Y., Besnard P., Abumrad N. A., and Khan N. A.. 2014. CD36- and GPR120-mediated Ca2+ signaling in human taste bud cells mediates differential responses to fatty acids and is altered in obese mice. Gastroenterology. 146: 995–1005. - PMC - PubMed
-
- Gilbertson T. A., and Khan N. A.. 2014. Cell signaling mechanisms of oro-gustatory detection of dietary fat: advances and challenges. Prog. Lipid Res. 53: 82–92. - PubMed
Publication types
MeSH terms
Substances
LinkOut - more resources
Full Text Sources
Medical
Miscellaneous