The TFAP2A-IRF6-GRHL3 genetic pathway is conserved in neurulation
- PMID: 30689861
- PMCID: PMC6494790
- DOI: 10.1093/hmg/ddz010
The TFAP2A-IRF6-GRHL3 genetic pathway is conserved in neurulation
Abstract
Mutations in IRF6, TFAP2A and GRHL3 cause orofacial clefting syndromes in humans. However, Tfap2a and Grhl3 are also required for neurulation in mice. Here, we found that homeostasis of Irf6 is also required for development of the neural tube and associated structures. Over-expression of Irf6 caused exencephaly, a rostral neural tube defect, through suppression of Tfap2a and Grhl3 expression. Conversely, loss of Irf6 function caused a curly tail and coincided with a reduction of Tfap2a and Grhl3 expression in tail tissues. To test whether Irf6 function in neurulation was conserved, we sequenced samples obtained from human cases of spina bifida and anencephaly. We found two likely disease-causing variants in two samples from patients with spina bifida. Overall, these data suggest that the Tfap2a-Irf6-Grhl3 genetic pathway is shared by two embryologically distinct morphogenetic events that previously were considered independent during mammalian development. In addition, these data suggest new candidates to delineate the genetic architecture of neural tube defects and new therapeutic targets to prevent this common birth defect.
Published by Oxford University Press 2019.
Figures
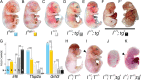
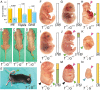
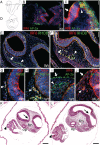
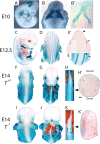
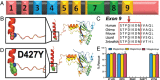
Similar articles
-
Tfap2a-dependent changes in mouse facial morphology result in clefting that can be ameliorated by a reduction in Fgf8 gene dosage.Dis Model Mech. 2015 Jan;8(1):31-43. doi: 10.1242/dmm.017616. Epub 2014 Nov 7. Dis Model Mech. 2015. PMID: 25381013 Free PMC article.
-
Shared molecular networks in orofacial and neural tube development.Birth Defects Res. 2017 Jan 30;109(2):169-179. doi: 10.1002/bdra.23598. Birth Defects Res. 2017. PMID: 27933721
-
Can a Liquid Biopsy Detect Circulating Tumor DNA With Low-passage Whole-genome Sequencing in Patients With a Sarcoma? A Pilot Evaluation.Clin Orthop Relat Res. 2025 Jan 1;483(1):39-48. doi: 10.1097/CORR.0000000000003161. Epub 2024 Jun 21. Clin Orthop Relat Res. 2025. PMID: 38905450
-
Impact of residual disease as a prognostic factor for survival in women with advanced epithelial ovarian cancer after primary surgery.Cochrane Database Syst Rev. 2022 Sep 26;9(9):CD015048. doi: 10.1002/14651858.CD015048.pub2. Cochrane Database Syst Rev. 2022. PMID: 36161421 Free PMC article. Review.
-
The effectiveness of abstinence-based and harm reduction-based interventions in reducing problematic substance use in adults who are experiencing homelessness in high income countries: A systematic review and meta-analysis: A systematic review.Campbell Syst Rev. 2024 Apr 21;20(2):e1396. doi: 10.1002/cl2.1396. eCollection 2024 Jun. Campbell Syst Rev. 2024. PMID: 38645303 Free PMC article. Review.
Cited by
-
Single-cell atlas of early chick development reveals gradual segregation of neural crest lineage from the neural plate border during neurulation.Elife. 2022 Jan 28;11:e74464. doi: 10.7554/eLife.74464. Elife. 2022. PMID: 35088714 Free PMC article.
-
Analysis of candidate genes for cleft lip ± cleft palate using murine single-cell expression data.Front Cell Dev Biol. 2023 Apr 24;11:1091666. doi: 10.3389/fcell.2023.1091666. eCollection 2023. Front Cell Dev Biol. 2023. PMID: 37169019 Free PMC article.
-
A Novel Van der Woude Syndrome-Causing IRF6 Variant Is Subject to Incomplete Non-sense-Mediated mRNA Decay Affecting the Phenotype of Keratinocytes.Front Cell Dev Biol. 2020 Sep 29;8:583115. doi: 10.3389/fcell.2020.583115. eCollection 2020. Front Cell Dev Biol. 2020. PMID: 33117810 Free PMC article.
-
Neuronal Migration Generates New Populations of Neurons That Develop Unique Connections, Physiological Properties and Pathologies.Front Cell Dev Biol. 2019 Apr 24;7:59. doi: 10.3389/fcell.2019.00059. eCollection 2019. Front Cell Dev Biol. 2019. PMID: 31069224 Free PMC article. Review.
-
In-frame deletion of SPECC1L microtubule association domain results in gain-of-function phenotypes affecting embryonic tissue movement and fusion events.Hum Mol Genet. 2021 Dec 17;31(1):18-31. doi: 10.1093/hmg/ddab211. Hum Mol Genet. 2021. PMID: 34302166 Free PMC article.
References
-
- Richardson R.J., Dixon J., Malhotra S., Hardman M.J., Knowles L., Boot-Handford R.P., Shore P., Whitmarsh A. and Dixon M.J. (2006) Irf6 is a key determinant of the keratinocyte proliferation–differentiation switch. Nat. Genet., 38, 1329–1334. - PubMed
Publication types
MeSH terms
Substances
Grants and funding
LinkOut - more resources
Full Text Sources
Molecular Biology Databases