Optical coherence tomography angiography: A comprehensive review of current methods and clinical applications
- PMID: 28760677
- PMCID: PMC5600872
- DOI: 10.1016/j.preteyeres.2017.07.002
Optical coherence tomography angiography: A comprehensive review of current methods and clinical applications
Abstract
OCT has revolutionized the practice of ophthalmology over the past 10-20 years. Advances in OCT technology have allowed for the creation of novel OCT-based methods. OCT-Angiography (OCTA) is one such method that has rapidly gained clinical acceptance since it was approved by the FDA in late 2016. OCTA images are based on the variable backscattering of light from the vascular and neurosensory tissue in the retina. Since the intensity and phase of backscattered light from retinal tissue varies based on the intrinsic movement of the tissue (e.g. red blood cells are moving, but neurosensory tissue is static), OCTA images are essentially motion-contrast images. This motion-contrast imaging provides reliable, high resolution, and non-invasive images of the retinal vasculature in an efficient manner. In many cases, these images are approaching histology level resolution. This unprecedented resolution coupled with the simple, fast and non-invasive imaging platform have allowed a host of basic and clinical research applications. OCTA demonstrates many important clinical findings including areas of macular telangiectasia, impaired perfusion, microaneurysms, capillary remodeling, some types of intraretinal fluid, and neovascularization among many others. More importantly, OCTA provides depth-resolved information that has never before been available. Correspondingly, OCTA has been used to evaluate a spectrum of retinal vascular diseases including diabetic retinopathy (DR), retinal venous occlusion (RVO), uveitis, retinal arterial occlusion, and age-related macular degeneration among others. In this review, we will discuss the methods used to create OCTA images, the practical applications of OCTA in light of invasive dye-imaging studies (e.g. fluorescein angiography) and review clinical studies demonstrating the utility of OCTA for research and clinical practice.
Keywords: Glaucoma; Macular degeneration; Optical coherence tomography angiography; Physiology; Retina; Vascular disease.
Copyright © 2017 Elsevier Ltd. All rights reserved.
Figures
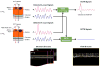
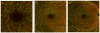
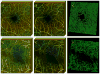
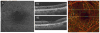
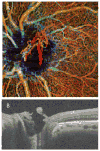
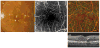
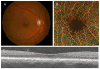
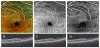
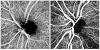
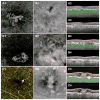
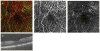
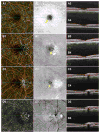
Similar articles
-
The application of optical coherence tomography angiography in retinal diseases.Surv Ophthalmol. 2017 Nov-Dec;62(6):838-866. doi: 10.1016/j.survophthal.2017.05.006. Epub 2017 Jun 1. Surv Ophthalmol. 2017. PMID: 28579550 Review.
-
The role of optical coherence tomography angiography in fundus vascular abnormalities.BMC Ophthalmol. 2016 Jul 13;16:107. doi: 10.1186/s12886-016-0277-2. BMC Ophthalmol. 2016. PMID: 27412442 Free PMC article.
-
Optical coherence tomography angiography: a review of the current literature.J Int Med Res. 2023 Jul;51(7):3000605231187933. doi: 10.1177/03000605231187933. J Int Med Res. 2023. PMID: 37498178 Free PMC article. Review.
-
[New Possibilities in Retinal Diagnostics Using OCT Angiography].Klin Monbl Augenheilkd. 2016 May;233(5):613-21. doi: 10.1055/s-0042-105325. Epub 2016 May 17. Klin Monbl Augenheilkd. 2016. PMID: 27187882 Review. German.
-
Optical coherence tomography angiography (OCTA) flow speed mapping technology for retinal diseases.Expert Rev Med Devices. 2018 Dec;15(12):875-882. doi: 10.1080/17434440.2018.1548932. Epub 2018 Nov 22. Expert Rev Med Devices. 2018. PMID: 30460869 Free PMC article. Review.
Cited by
-
Trends and hotspots in the field of diabetic retinopathy imaging research from 2000-2023.Front Med (Lausanne). 2024 Oct 9;11:1481088. doi: 10.3389/fmed.2024.1481088. eCollection 2024. Front Med (Lausanne). 2024. PMID: 39444814 Free PMC article.
-
Robust three-dimensional registration on optical coherence tomography angiography for speckle reduction and visualization.Quant Imaging Med Surg. 2021 Mar;11(3):879-894. doi: 10.21037/qims-20-751. Quant Imaging Med Surg. 2021. PMID: 33654662 Free PMC article.
-
Severity Stratification of Coronary Artery Disease Using Novel Inner Ellipse-Based Foveal Avascular Zone Biomarkers.Invest Ophthalmol Vis Sci. 2024 Oct 1;65(12):15. doi: 10.1167/iovs.65.12.15. Invest Ophthalmol Vis Sci. 2024. PMID: 39382880 Free PMC article.
-
Towards distortion-free imaging of the eye.PLoS One. 2021 Jun 10;16(6):e0252876. doi: 10.1371/journal.pone.0252876. eCollection 2021. PLoS One. 2021. PMID: 34111195 Free PMC article.
-
Radiomics in ophthalmology: a systematic review.Eur Radiol. 2025 Jan;35(1):542-557. doi: 10.1007/s00330-024-10911-4. Epub 2024 Jul 21. Eur Radiol. 2025. PMID: 39033472 Review.
References
-
- Classification of diabetic retinopathy from fluorescein angiograms. ETDRS report number 11. Early Treatment Diabetic Retinopathy Study Research Group. Ophthalmology. 98(5 Suppl):807–822. - PubMed
-
- Adhi M, Filho MA, Louzada RN, Kuehlewein L, de Carlo TE, Baumal CR, Witkin AJ, Sadda SR, Sarraf D, Reichel E, Duker JS, Waheed NK. Retinal Capillary Network and Foveal Avascular Zone in Eyes with Vein Occlusion and Fellow Eyes Analyzed With Optical Coherence Tomography Angiography. Invest Ophthalmol Vis Sci. 2016;57(9):OCT486–494. - PubMed
-
- Adhi M, Filho MAB, Louzada RN, Kuehlewein L, de Carlo TE, Baumal CR, Witkin AJ, Sadda SR, Sarraf D, Reichel E, Duker JS, Waheed NK. Retinal Capillary Network and Foveal Avascular Zone in Eyes with Vein Occlusion and Fellow Eyes Analyzed With Optical Coherence Tomography Angiography. Investigative Ophthalmology & Visual Science. 2016;57(9):OCT486–489. - PubMed
-
- Agemy SA, Scripsema NK, Shah CM, Chui T, Garcia PM, Lee JG, Gentile RC, Hsiao Y-S, Zhou Q, Ko T, Rosen RB. RETINAL VASCULAR PERFUSION DENSITY MAPPING USING OPTICAL COHERENCE TOMOGRAPHY ANGIOGRAPHY IN NORMALS AND DIABETIC RETINOPATHY PATIENTS. Retina (Philadelphia, Pa) 0002;35(11):1–11. - PubMed
-
- Agemy SA, Scripsema NK, Shah CM, Chui T, Garcia PM, Lee JG, Gentile RC, Hsiao YS, Zhou Q, Ko T, Rosen RB. Retinal Vascular Perfusion Density Mapping Using Optical Coherence Tomography Angiography in Normals and Diabetic Retinopathy Patients. Retina. 2015;35(11):2353–2363. - PubMed
Publication types
MeSH terms
Grants and funding
LinkOut - more resources
Full Text Sources
Other Literature Sources
Medical
Miscellaneous