Smad3 recruits the anaphase-promoting complex for ubiquitination and degradation of SnoN
- PMID: 11691834
- PMCID: PMC312804
- DOI: 10.1101/gad.912901
Smad3 recruits the anaphase-promoting complex for ubiquitination and degradation of SnoN
Abstract
Smad proteins mediate transforming growth factor-beta (TGF-beta) signaling to regulate cell growth and differentiation. SnoN is an important negative regulator of TGF-beta signaling that functions to maintain the repressed state of TGF-beta target genes in the absence of ligand. On TGF-beta stimulation, Smad3 and Smad2 translocate into the nucleus and induce a rapid degradation of SnoN, allowing activation of TGF-beta target genes. We show that Smad2- or Smad3-induced degradation of SnoN requires the ubiquitin-dependent proteasome and can be mediated by the anaphase-promoting complex (APC) and the UbcH5 family of ubiquitin-conjugating enzymes. Smad3 and to a lesser extent, Smad2, interact with both the APC and SnoN, resulting in the recruitment of the APC to SnoN and subsequent ubiquitination of SnoN in a destruction box (D box)-dependent manner. In addition to the D box, efficient ubiquitination and degradation of SnoN also requires the Smad3 binding site in SnoN as well as key lysine residues necessary for ubiquitin attachment. Mutation of either the Smad3 binding site or lysine residues results in stabilization of SnoN and in enhanced antagonism of TGF-beta signaling. Our studies elucidate an important mechanism and pathway for the degradation of SnoN and more importantly, reveal a novel role of the APC in the regulation of TGF-beta signaling.
Figures
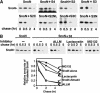
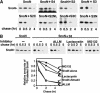
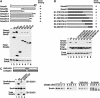
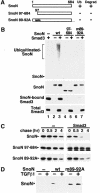
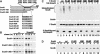
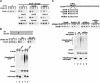
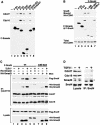
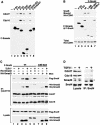
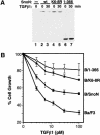
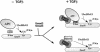
Similar articles
-
NEDD4-2 (neural precursor cell expressed, developmentally down-regulated 4-2) negatively regulates TGF-beta (transforming growth factor-beta) signalling by inducing ubiquitin-mediated degradation of Smad2 and TGF-beta type I receptor.Biochem J. 2005 Mar 15;386(Pt 3):461-70. doi: 10.1042/BJ20040738. Biochem J. 2005. PMID: 15496141 Free PMC article.
-
The anaphase-promoting complex mediates TGF-beta signaling by targeting SnoN for destruction.Mol Cell. 2001 Nov;8(5):1027-39. doi: 10.1016/s1097-2765(01)00382-3. Mol Cell. 2001. PMID: 11741538
-
Cytoplasmic SnoN in normal tissues and nonmalignant cells antagonizes TGF-beta signaling by sequestration of the Smad proteins.Proc Natl Acad Sci U S A. 2005 Aug 30;102(35):12437-42. doi: 10.1073/pnas.0504107102. Epub 2005 Aug 18. Proc Natl Acad Sci U S A. 2005. PMID: 16109768 Free PMC article.
-
[Role of Ski/SnoN protein in the regulation of TGF-beta signal pathway].Zhongguo Yi Xue Ke Xue Yuan Xue Bao. 2003 Apr;25(2):233-6. Zhongguo Yi Xue Ke Xue Yuan Xue Bao. 2003. PMID: 12905729 Review. Chinese.
-
[Ski and SnoN: antagonistic proteins of TGFbeta signaling].Bull Cancer. 2000 Feb;87(2):135-7. Bull Cancer. 2000. PMID: 10705283 Review. French.
Cited by
-
Developmental defects observed in hypomorphic anaphase-promoting complex mutants are linked to cell cycle abnormalities.Development. 2003 Apr;130(8):1605-20. doi: 10.1242/dev.00385. Development. 2003. PMID: 12620985 Free PMC article.
-
APC/CCdh1 regulates the balance between maintenance and differentiation of hematopoietic stem and progenitor cells.Cell Mol Life Sci. 2019 Jan;76(2):369-380. doi: 10.1007/s00018-018-2952-3. Epub 2018 Oct 24. Cell Mol Life Sci. 2019. PMID: 30357422 Free PMC article.
-
APC/C-Cdh1: from cell cycle to cellular differentiation and genomic integrity.Cell Cycle. 2010 Oct 1;9(19):3904-12. doi: 10.4161/cc.9.19.13585. Epub 2010 Oct 11. Cell Cycle. 2010. PMID: 20935501 Free PMC article. Review.
-
The anaphase-promoting complex coordinates initiation of lens differentiation.Mol Biol Cell. 2007 Mar;18(3):1018-29. doi: 10.1091/mbc.e06-09-0809. Epub 2007 Jan 10. Mol Biol Cell. 2007. PMID: 17215516 Free PMC article.
-
Arsenite-induced Cdc25C degradation is through the KEN-box and ubiquitin-proteasome pathway.Proc Natl Acad Sci U S A. 2002 Feb 19;99(4):1990-5. doi: 10.1073/pnas.032428899. Epub 2002 Feb 12. Proc Natl Acad Sci U S A. 2002. PMID: 11842186 Free PMC article.
References
-
- Akiyoshi S, Inoue H, Hanai J, Kusanagi K, Nemoto N, Miyazono K, Kawabata M. c-Ski acts as a transcriptional co-repressor in transforming growth factor-beta signaling through interaction with smads. J Biol Chem. 1999;274:35269–35277. - PubMed
-
- Bonni S, Wang H-R, Causing CG, Kavsak P, Stroschein SL, Luo K, Wrana JL. TGF-β induces assembly of a Smad2–Smurf2 ubiquitin ligase complex that targets SnoN for degradation. Nat Cell Biol. 2001;3:587–595. - PubMed
-
- Boyer PL, Colmenares C, Stavnezer E, Hughes SH. Sequence and biological activity of chicken snoN cDNA clones. Oncogene. 1993;8:457–466. - PubMed
-
- Catzavelos C, Bhattacharya N, Ung YC, Wilson JA, Roncari L, Sandhu C, Shaw P, Yeger H, Morava-Protzner I, Kapusta L, et al. Decreased levels of the cell-cycle inhibitor p27Kip1 protein: Prognostic implications in primary breast cancer. Nat Med. 1997;3:227–230. - PubMed
Publication types
MeSH terms
Substances
Grants and funding
LinkOut - more resources
Full Text Sources
Other Literature Sources
Molecular Biology Databases
Miscellaneous